Optimizing a greenhouse to maximize crop productivity and resource efficiency can be a tricky project to undertake. Dynamic production factors such as sunlight and outdoor climate (i.e. temperature and humidity) make these structures more difficult to control compared to their indoor farm counterparts.
For example, greenhouse operators must design their facilities with the worst-case-scenario conditions in mind (e.g. during northern region winters), leading to the integration of expensive supplemental lighting systems. In a bid to justify the equipment’s purchase, some operators may elect to use those lights to maximize light intensity over the entire day, no matter the amount of sunlight. While this approach can have its benefits, it can also lead to higher energy costs and crop stress when leaf temperatures become excessive or irrigation systems are not able to keep up with transpiration rates.
Growers might also choose to activate lighting based on solar light intensity. A solar intensity-focused control program would activate lights during the defined photoperiod if the photosynthetic photon flux density (PPFD) from sunlight dropped below 400 µmols/m2/s inside the greenhouse until it reached 500 µmols/m2/s. If the light sunlight reached 1,200 µmols/m2/s, the shade curtains would close to reduce cooling load and plant stress. The shade curtains would retract when the light intensity dropped to 900 µmols/m2/s to maintain optimal growth parameters. Purdue University found that controlling lighting based on solar intensity reduced energy consumption by 30%.
What is PPFD?
From RII’s Lighting Best Practices Guide: “Photosynthetic Photon Flux Density (PPFD), is the quantity of photons within the PAR range, measured in micromoles, that hit a given area (a square meter) of your plant canopy per second.”
Plan ahead
Rather than target a fixed photosynthetic photon flux density (the number of photosynthetically active photons hitting a one-square-meter area per second, or PPFD for short), greenhouse growers can build automated systems around daily light integral (DLI). DLI is the measure of the amount of photosynthetically active photons delivered into a certain area over 24 hours. In other words, this rolling average metric multiplies the PPFD levels with the photoperiod to quantify the amount of light a plant receives in a day. Think of it as a rain gauge, only for photons of light rather than drops of water.
Researchers have determined the optimum DLI for nearly all of the crop species used in CEA operations. For example, lettuce has an optimum DLI of 12-17 mol/day, so greenhouse lighting could be programmed to shut off for the rest of the day once 17 mol/day is reached. From the example above, this DLI could be achieved in a minimum of 4 hours on a clear day and a maximum of 10 hours on a cloudy day, meaning that a potential 6 hours of lighting could be saved, along with the corresponding energy savings.
“DLI is a measurement of light on the plants’ terms,” says Loren West, CTO and founder at RII member Microclimates Inc., a manufacturer of environmental control systems software. By ignoring DLI, West warns both indoor and greenhouse growers will not be able to have an efficient cultivation process and waste resources and capital. “If [plants are] not getting exactly what they need, you’re overspending. If they’re not getting enough, your plant health is at risk,” he notes.
By tracking and building systems around DLI, operators can build a more resilient system that better manages greenhouse input variances.
Approaching DLI in CEA Production
Due to the time element in DLI’s calculations, it can be difficult for facility operators to make production decisions around this metric without robust data collection and analysis. Should supplemental lights be turned on at 20% or 50% capacity when there is a 2-hour cloudy period in the morning? Should shade curtains be drawn on the fifth day of full sun, or should crops be left to soak in the extra sunlight?
Before trying to fine-tune a greenhouse based on DLI, it’s important to understand each crop’s DLI needs and limits, says Neda Vaseghi, Microclimates’ CEO and co-founder. Still today, many greenhouse operators make production decisions based on incomplete data or even their gut feelings, she explains, noting how some growers “just turn [their] lights on, cross [their] fingers and hope there’s enough light for the crop.”
While that approach can work, “what it’s not doing is optimizing the potential cost savings from an energy perspective, and it’s not optimizing the growth of the plant of that particular crop.” (See Table 1 for a sample of DLI targets for some of the most popular greenhouse-grown food and medicinal crops)
Table 1: DLI targets by crop type
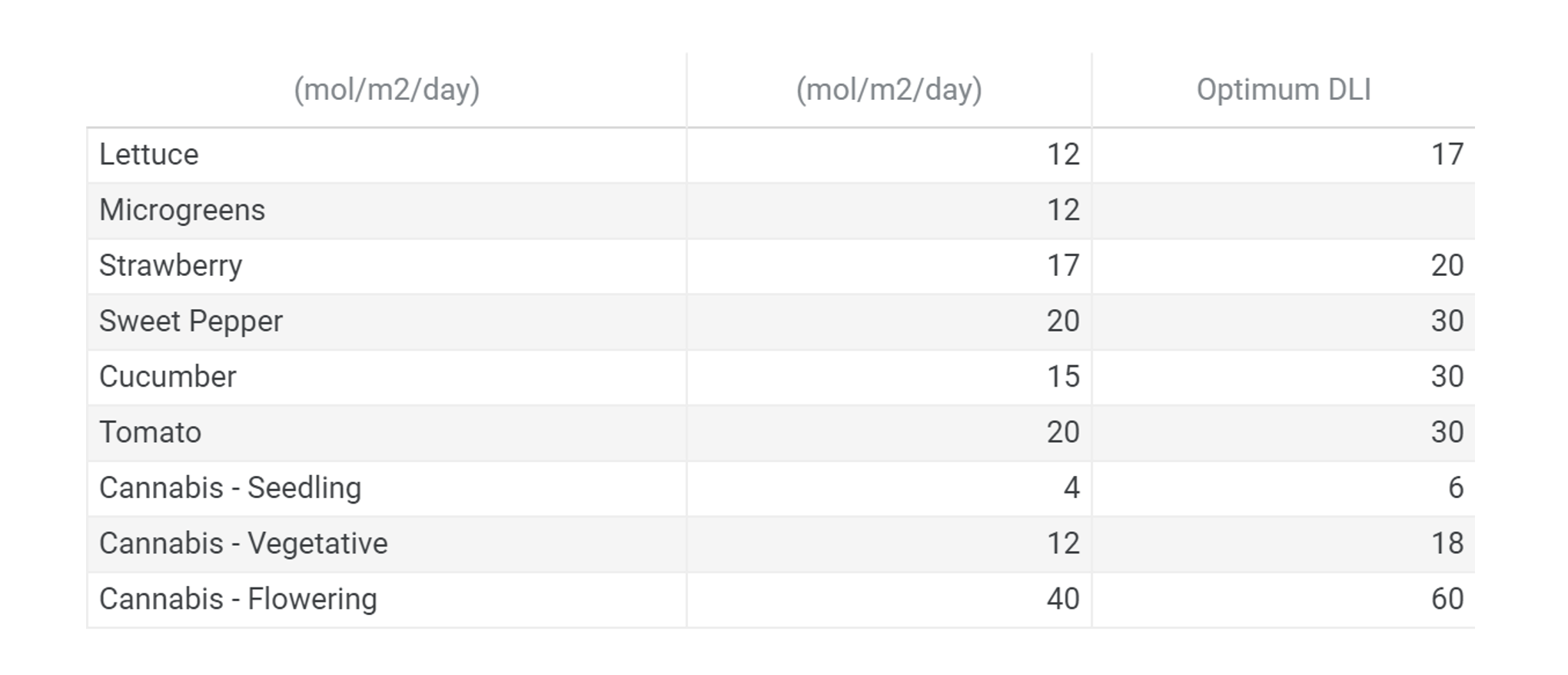
Crop data by Dr. Neil Mattson, Director of Cornell University Controlled Environment Agriculture group. Cannabis data aggregated from multiple sources by Microclimates.
Once greenhouse operators establish the crops’ ideal DLI targets, they can begin tracking light levels throughout their structure to gain a granular view of their facility’s light potential on the worst, average, and best days. Greenhouse design, geographies, and weather patterns will influence the amount of light reaching crops, sometimes combining to create light microclimates. “Knowing your microclimates of light inside of your greenhouse is just as important as knowing your microclimates of climate,” West says.
Vaseghi suggests that each cultivation zone has more than one light sensor. By taking the average reading from multiple sensors, operators can ensure lights do not turn off and on as shadows move across the zone. If only a single PAR sensor is available, programming a delayed response to light shifts–for example by taking the average of a 15-minute reading window–can also avoid excessive equipment use.
Predictive DLI’s Energy Saving & Productivity Potential
With the right combinations of data collection ability and smart controls, predictive DLI-based analytics can help greenhouses decrease energy usage by up to 30%, West and Vaseghi say. That’s because predictive DLI control systems use the collected data to make prescriptive predictions and adjust the environment to hit a rolling average target rather than specific daily metrics.
For example, a greenhouse using a DLI system set to make decisions based on the last five days’ averages might increase the amount of supplemental lighting because of the recent three-day cloudy period, maintaining the crop’s optimal productivity. Vice versa, passive cooling systems such as shade curtains might be activated and supplemental lighting completely turned off after a particularly sunny period, saving on energy costs.
Put simply, the carryover inputs from the previous period influence the application of those inputs in the future. “Sometimes, if it’s really dark, greenhouses won’t have enough lights to produce that DLI that day,” West explains. “You want a 10 [DLI], but because of today’s clouds, you’re only getting a 9. 5 or 9. 2. So your target [DLI] is higher [the next day]. Over time, the Sun’s going to come back. A smart DLI program is going to take advantage of the fact that the next few days the Sun really did come out and is going to be able to make up for those losses.”
DLI-based system vendors often have preset conditions programmed, but those can often be tweaked or replaced wholesale to fit the CEA facility’s specific needs. Cornell University’s Greenhouse Lighting and Systems Engineering (GLASE) consortium’s Light and Shade System Implementation (LASSI) algorithm is publicly available code that schedules supplemental lighting and shade to complement sunlight to maintain a constant DLI and does so while prioritizing off-peak lighting use to minimize energy expenses. LASSI can be implemented into any DLI system (Microclimates Inc. is in the process of integrating this code into its DLI control system to help with the commercialization of this code in North American greenhouses).
In 2020, Cornell released a technical paper showing how integrating CO2 control into the LASSI algorithm could reduce the hours of supplemental lighting needed by up to 27% per year in the Central New York region, again demonstrating the power of evolving smart systems and prescriptive analytics.
DLI systems can also have benefits in indoor farms, although the greater control of lighting and environmental conditions limits their efficiency potential. However, light fixtures can degrade over time, and power fluctuations during the day can lead to the fixtures’ PPFD output being lower than what the system is rated. By monitoring DLI, control systems can adjust environmental settings to account for those input losses, or can warn the operator that maintenance is needed.
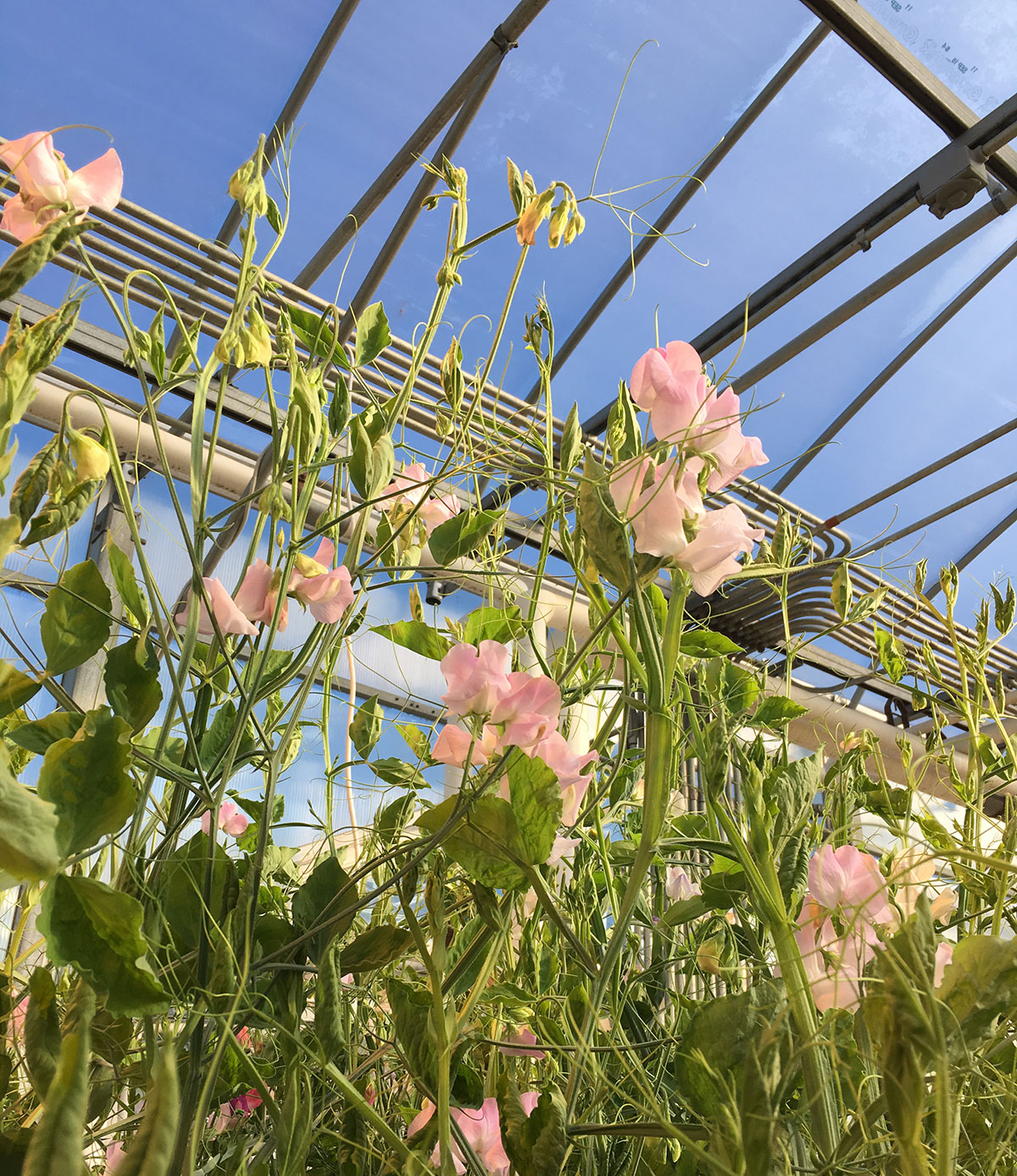
West stresses the importance of monitoring light intensities at the crop level and adds that doing so can also help discover utility-related issues, such as a lack of stable power coming to the facility. “If you’re not reading [crop-level lighting data], you’re not seeing that [light levels] … dip at this time of day. You wouldn’t ever expect it if you’re not reading it. And it may just be that your lights are getting a little bit less power from two o’clock to four o’clock because this shop next door is sucking some of your energy.”
The key to navigating the complexities of optimizing greenhouse productivity can be found in a more adaptive strategy centered around predictive DLI. Embracing predictive DLI represents a shift in greenhouse management, where cultivation decisions are guided by a holistic understanding of light requirements. As technology advances and smart systems become more sophisticated, the integration of predictive DLI-based approaches promises not only increased resource efficiency but also improved crop health and yield. By acknowledging and adapting to the dynamic nature of light in plant growth, CEA operators can unlock the full potential of their cultivation endeavors, paving the way for sustainable and optimized agricultural practices in the future.
Meet Our Members
Thank you to our members who participated in this blog. Read more about their organizations below:
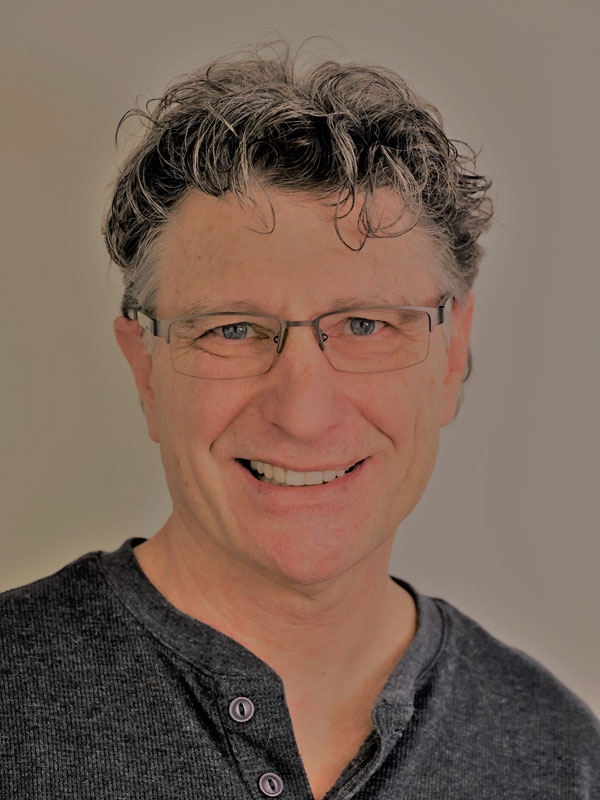
Loren West is the CTO and Founder at Microclimates, a software company in controlled environment agriculture (CEA), supporting indoor farming practices. He is a team leader with an accomplished background directing development of leading-edge software products from concept to delivery. He has a broad understanding of technology from small footprint mobile devices through big data server deployments and specializes in enterprise and consumer software products in Node.js, JavaScript, ObjectiveC, Java, and open source.
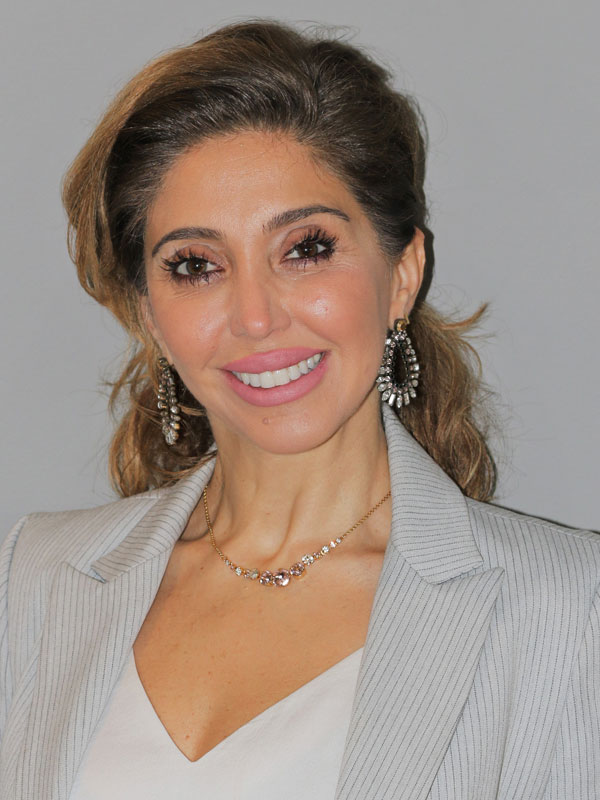
Neda Vaseghi is the CEO and co-founder of Microclimates, a software company in controlled environment agriculture (CEA). Neda is responsible for running all facets of the business. She has a proven track record of managing teams and more than 25 years of experience driving business growth. Prior to Microclimates, she held various roles at Merieux NutriSciences, a global food safety company, where she was responsible for North America Mergers & Acquisition. Her passion for agriculture and sustainability started early on at the University of Washington School of Public Health. Neda holds a Bachelor of Science & MBA.